Research
Scalable and programmable atom arrays are a fast-rising platform for quantum simulation, quantum computation, and quantum metrology. They offer exquisite control over many degrees of freedom, including the internal quantum states, motional states, array geometries, and doping. By driving the atoms to highly excited Rydberg states, the atoms can be made to interact over long range. The interacting atoms can in turn be exploited for many applications like simulating quantum magnetism and executing quantum logic gates.
Our laboratory is interested in using atom arrays to process quantum information and to simulate advanced materials. To date, we have developed tools to scale up and enhance the programmability of atom arrays. Our key results are summarized below.
Floquet-tailored Rydberg interactions
Quantum processors generally benefit from increased qubit connectivity, improved coherence, and new state-initialization pathways. We show that neutral atom quantum processors can be advanced on all three critical fronts through a simple, robust approach – Floquet frequency modulation:
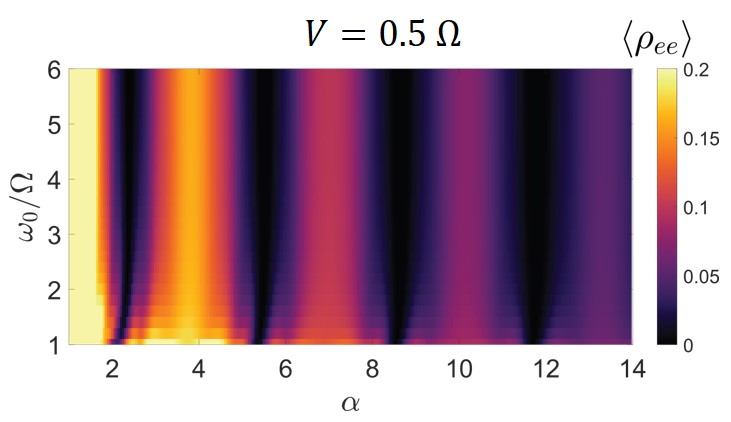
- We demonstrate Rydberg-blockade entanglement beyond the conventional blockade radius for two tweezer-trapped atoms and showed how the enlarged entanglement range improves qubit connectivity in a neutral atom array.
- We find that the coherence of entangled states can be protected against Doppler dephasing, which is the dominant mechanism limiting entangled-state coherence in atom arrays.
- We propose a robust method to realize Rydberg anti-blockade states in the steady state for two closely-spaced atoms, which cannot be otherwise achieved with a conventional static drive.
Scaling up to large defect-free atom arrays
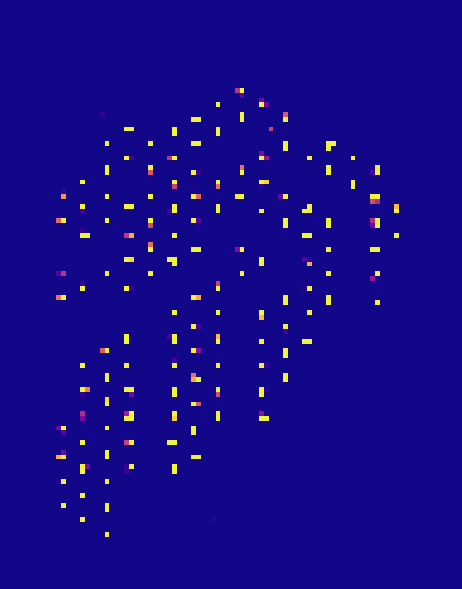
We realize large pristine arrays containing hundreds of atoms with high success rates by using a new algorithm that moves multiple atoms at the same time. Defect-free arrays of atoms are an important platform for quantum simulation and quantum computing, and large arrays allow us to harness more atoms for increased simulation and computing power. However, producing large defect-free arrays can be challenging because of the losses encountered during assembly. One way to beat the losses is to build the arrays as quickly as possible. Our new algorithm achieves a significant speedup over existing algorithms by using multiple tweezers to sort atoms in parallel. We have also used our new algorithm to create a range of pristine patterns including a honeycomb and a lion head.
Phys. Rev. Applied publication, APS Physics highlight, NUS news highlight, Phys.org, CQT highlight
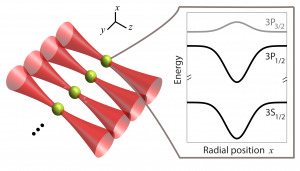
Scaling up atom arrays with magic wavelength tweezers
We use a new class of magic wavelengths (for the D1 transition in alkalis) to achieve an order-of-magnitude reduction in the optical power required per tweezer trap, which allows us to scale up the atom array size correspondingly.
Read more about it:
The big deal about small building blocks (for the layman)
Previous research on cold and ultracold molecules in bulk gases (for the expert)